Selected Major Results: Stars and Planetary Systems
- The PLATO Space Mission
- Superflares
- Determining the masses of non-transiting exoplanets in multi-planetary systems
- Planets around metal-rich stars are less suitable for life
The PLATO Space Mission
PLATO is the third medium-class space mission in the Cosmic Vision 2015-2025 Program of the European Space Agency (ESA) and it will be launched in 2026. The Solar and Stellar Interiors Department at the Max Planck Institute for Solar System Research (MPS) is the host of the PLATO Data Center DataBase.
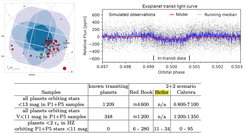
Within the PLATO Data Center, MPS has the responsibility to implement the data pipelines that are parts of the PLATO science ground segment that will be run at ESA's Science Operations Center. At MPS, we also produce the relevant documentation according to the PLATO document tree and we support the validation and verification of the software. The MPS also contributes to the PLATO Data Center DataBase, which acts as a central hub for data exchange for the entire PLATO Mission Consortium throughout Europe. We also develop the PLATO Data Center system architecture, the Data Analysis Support Tools for the validation of PLATO data products , algorithms for on-board data processing, and we contribute to the PLATO Input Catalog that contains hundreds of thousands of stellar targets for PLATO and millions of contaminants.
Researchers at the PLATO Data Center at MPS also support the science activities of PLATO. During the first four years of its long-duration observation phase, PLATO will observe two large fields in the sky (see Figure for the northern pointing). With PLATO's major science goal of detecting Earth-sized planets in the habitable zone around Sun-like stars in mind, our simulated PLATO observations and predictions of exoplanet yield rates (Heller et al. 2022) can help to make informed decisions on the choice of the duration of the observation fields. Learn more about the PLATO mission
References:
- R. Heller, J.-V. Harre, R. Samadi (2022) Astron. Astrophys. 665, A11 [doi]
- M. Kidger, C. Damiani, C. Martín, A. Labiano (2023) PLATO Level 1 Subsystem Design Document [cosmos]
- C. Martín, A. Labiano, C. Damiani and D. Sánchez (2023) PLATO CPDS Sub-System Requirements and Design Document [cosmos]
- V. Nascimbeni et al. (2022) Astron. Astrophys. 658, A31 [doi]
- H. Rauer, et al. including MPS team (2023) PLATO review paper to be submitted
Press Release and Media Appearances:
- Press Release article
- "Max-Planck-Institut für Sonnensystemforschung: Team entdeckt neue Schwingungen der Sonne" Göttinger Tageblatt (in German)
- Finding new Earths: PLATO spacecraft to be built, June 2017
Superflares
The detection of superflares is challenging due to potential sources of contamination, such as background stars or small solar system objects appearing in the same aperture as the primary target.
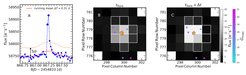
In Vasilyev et al. (2022) we presented a new method for identifying the true flare sources in large photometric surveys using data from the Kepler mission as an example. First, we searched the light curves for potential flares (see figure). In the second step, we used a Bayesian approach to fit the point spread function of the instrument to determine the most likely location of the flux excess on the detector (see figure panels B and C). We applied our method to 5862 main-sequence stars with near-solar effective temperatures and detected 342 superflares. Of these, 283 flares occurred on 178 target stars, 47 events were associated with fainter background stars, and in 10 cases, the flare location could not be distinguished between the target and a background star. To understand how often superflares occur on solar-like stars we applied our analysis to a sample of ∼59000 Kepler stars and detected 3524 flares. Detailed results, including the flare energy distribution and their occurrence rates, will be presented in a forthcoming paper.
References
- S. Okamoto, Y. Notsu, H. Maehara, et al. (2021), Astrophys. J. 906, 72
- T. Shibayama, H. Maehara, S. Notsu, et al. (2013), Astrophys. J. Suppl. 209, 5
- V. Vasilyev, T. Reinhold, A.I. Shapiro, et al. (2022), Astron. Astrophys. 668, A167
Determining the masses of non-transiting exoplanets in multi-planetary systems
In addition to the planetary atmospheric composition, a key parameter for the formation of life (as we know it) is whether the planet has a rocky or a gaseous structure. Accurate masses of habitable zone planets orbiting low mass stars have so far only been determined using a combination of transit and RV observations. Provided the stellar mass is known, the RV observations alone can only provide a minimum planetary mass, mp sin ip, where ip is the angle between the orbital plane and the plane of the sky. However, none of the planets within 5 pc are known to be transiting.
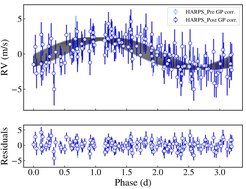
Jeffers and Dreizler have developed a new method to determine the true planetary masses of non-transiting multi-planetary systems orbiting in the habitable zones of low mass stars. This method uses N-body integrations to determine the mutual gravitational interaction between the planets in a multi-planetary system in compact orbits. This breaks the degeneracy between planetary mass and inclination, and ultimately gives the true mass of each individual planet.
The close-by star GJ1061 has three Earth-mass planets previously discovered by the RedDots team (Dreizler et al. 2020) with periods of 3.2, 6.7 and 13 days and which are close to the 1:2:4 period commensurability. The outermost planet lies in GJ1061’s liquid water habitable zone and receives a similar amount of energy as the Earth does from the Sun. The results from the mutual gravitational interaction indicate that the two outer planets are coplanar and that the inner planet has an inclination of about 15 degrees. We can also confirm that the outer planet has a rocky Earth-mass internal composition rather than a more gaseous composition that would be expected for a slightly heavier sub-Neptunian planet.
References:
- S. Dreizler, S. Jeffers, et al. (2020) MNRAS 493, 536 [ads]
- S. Jeffers, et al. (2020) Science 368, 1477 [doi]
- S. Jeffers, S. Dreizler, & the RedDots team (2023), Poster presented at the conference "Towards Other Earths III: The Planet-Star connection", 17-21 July 2023, Porto [doi]
Planets around metal-rich stars are less suitable for life
In Anna Shapiro et al. (2023) we address the intriguing question of what would happen to the ozone layer if the Earth were hosted by another Sun-like star. We modeled the atmosphere of an exo-Earth with a 1D radiative-convective model developed at the Max Planck Institute for Chemistry in Mainz and calculated O3 concentration and surface UV as functions of stellar UV radiation levels (via the stellar effective temperature and stellar metallicity). To do this we used stellar spectra corresponding to near-solar effective temperatures (from 5300 to 6300 K) and metallicity in the range of ±1 dex relative to the Sun. The spectra were obtained using the latest MPS-ATLAS code (Kostogryz et al. 2022).
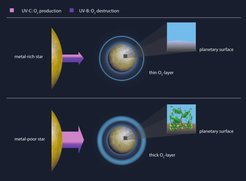
Our study shows that, paradoxically, although metal-rich stars emit less UV radiation than metal-poor stars, the drop in ozone concentration leads to higher surface UV on the planets (see figure). This implies that the Universe becomes less friendly to life as it ages because stellar metallicity increases.
We also estimated the impact of internal and external catastrophic events such as sudden increases in stellar magnetic activity, supernovae explosion, and volcanic eruptions on the surface radiative stress. Our study shows that while volcanic eruptions are the most ozone destructive hazards, oxidized atmospheres (O2 level higher than 20%) have sufficient buffering capacity to limit the damage. Moreover, our calculations indicate that recently proposed events of sudden rise of stellar magnetic activity (Reinhold et al. 2020) results in increased ozone concentration and drop in surface UV.
References:
- N.M. Kostogryz, et al. (2022), Astron. Astrophys. 666, A60 [doi]
- T. Reinhold, et al. (2020), Science 368, 518 [doi]
- Anna V. Shapiro, C. Bruehl, K. Klingmueller, B. Steil, A.I. Shapiro, V. Witzke, N.M. Kostogryz, L. Gizon, S.K. Solanki, & J. Lelieveld (2023), Nature Comm. 14, 1893 [doi]
- Calibrating the stellar mixing-length parameter
- Classification of active red giants
- Butterfly diagrams of Kepler stars are unlike that of the Sun
- Discovering small planets using a new transit detection algorithm
- Distant star is roundest object ever observed in nature (2016)
- Interior Rotation of a Distant Star Revealed (2013)