Archived Major Results: Stars and Planetary Systems
- Calibrating the stellar mixing-length parameter
- Classification of active red giants
- Butterfly diagrams of Kepler stars are unlike that of the Sun
- Discovering small planets using a new transit detection algorithm
From older Research Highlights:
- Distant star is roundest object ever observed in nature (2016)
- Interior Rotation of a Distant Star Revealed (2013)
Calibrating the stellar mixing-length parameter
In the outer layers of Sun-like stars (M < 1.3 M⊙), the large radiative opacity triggers the transport of energy by convection. The presence of a convective envelope greatly affects the interior stratification of the star and, in turn, its surface radius. To construct accurate models of Sun-like stars thus requires a sufficiently realistic treatment of convective regions. This is a long-standing problem of stellar physics, since no self-consistent theoretical description of convection exists to date.
Standard stellar evolution codes usually rely on the mixing length theory (MLT) to model convective layers. In this prescription, the overall efficiency of convective energy transport is controlled by a free parameter, aMLT, which requires external calibration. Most commonly, this parameter is either freely adjusted, or set using the Sun as a calibrator, exploiting the fact that its mass, radius, and age are independently and accurately known. The implicit assumption of this "solar calibration", namely, that the MLT parameter can be kept constant independently of the mass, chemical composition, and evolutionary status of the star, is however theoretically unfounded and unsupported by observations.
Spada & Demarque (2019) demonstrate the use of a novel approach to the MLT calibration, first implemented by Spada et al. (2018). In this technique, the value of aMLT in the 1D stellar evolution code is calibrated to match the adiabatic specific entropy derived from 3D radiation-hydrodynamics simulations of stellar convective envelopes, whose effective temperature, surface gravity, and metallicity are selected consistently along the evolutionary track. This "entropy calibration" effectively removes the freedom in choosing aMLT, and naturally endows it with a dependence on the surface conditions of the star (i.e. Teff, log g, metallicity) at each evolutionary time step.
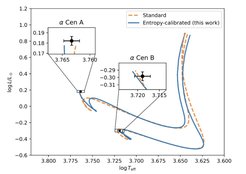
We compared the results of the standard and the entropy calibration in modeling the stars α Centauri A and B. These stars are ideal targets for such a test, since their parameters are known with very high accuracy. In addition, previous studies have shown that their measured radii cannot be reproduced by models evolved with the solarcalibrated value of aMLT, but require ad-hoc tuning of this parameter.
Our results are illustrated in Figure. Although the entropy-calibrated models contain one less degree of freedom with respect to the standard ones, in which aMLT is freely adjusted, their level of agreement with the observational constraints is equally satisfactory (within approximately 2σ, or better). The residual discrepancy can be ascribed to the intrinsic uncertainty associated with the entropy extracted from the numerical simulations of convection, as well as other modeling uncertainties, not related to convection.
[Go back to Research Topic and Recent Result, or to the top of this page ]
References:
- Spada, F., Demarque, P., Mon. Not. R. Astron. Soc. 489, 4712 (2019).
- Spada, F., Demarque, P., Basu, S., Tanner, J. D., Astrophys. J. 869, 135 (2018).
Classification of active red giants
During its evolution along the red-giant branch (RGB), a star like the Sun considerably expands (up to 100 times) before its radius reaches about 10 R⊙ and helium fusion starts in the core (red clump phase). Red giant stars all have a small core surrounded by a very large convective envelope, regardless of their masses.
In general, stars with convective envelopes can develop global magnetic fields that form starspots. According to dynamo theory, a star will efficiently develop magnetic fields when its rotation period is shorter than its convective turnover time. Besides causing spots on stellar surfaces, magnetic fields play a role in angular momentum evolution. At the end of the main sequence phase of their evolution, stars with convective envelopes have lost much of their initial spin.
The objective of the work by Gaulme et al. (2020) was to reassess the fraction of red giants that are magnetically active and to understand why. One of the underlying questions was the role of stellar binarity: red giants in close binary systems are spun up by tidal forces and have large starspots (Gaulme et al. 2014). Gaulme et al. (2020) make use of a combination of space-based photometric and ground-based spectroscopic data to classify the stars. Photometric data are used to measure stellar rotation rates (spots modulate the light curves), as well as the stellar mass, radius, and evolutionary stage (asteroseismology). Spectroscopic data are used to determine stellar binarity by tracking periodic Doppler shifts in the absorption spectra.

Figure. The three groups of rapidly rotating red-giant stars classified by Gaulme et al. (2020). © MPS / hormesdesign.de
Based on a sample of 4500 red giants observed by the Kepler satellite, Gaulme et al. (2020) determined that 99.3% of the stars are oscillating and that 8% have spots (instead of the previously reported 2%). They also measured the evolutionary stage (RGB vs. red clump) of more than 3000 of these stars. From a subset of 85 active red giants for which radial velocity data is available, about 15% appear to belong to close binary systems, which is significantly more than regular red giants (1%). The remaining 85% of active red giants are single stars and can be split into two groups: a lowmass (M ≤ 1.5 M⊙) and an intermediate-mass range (1.8 M⊙ ≤ M ≤ 2.8 M⊙). In the low-mass group, there are more active stars on the red clump than on the RGB, which means that a fraction of red giants gain angular momentum during the RGB. This supports the scenarios of planet engulfment or merger with small stellar objects. In the intermediate-mass group, we observe the opposite trend: RGB stars are more active than red clump stars, suggesting that angular momentum is lost during evolution. Figure summarizes these results.
[Go back to Research Topic and Recent Result, or to the top of this page ]
References
- Gaulme, P., Jackiewicz, J., Spada, F., et al., Astron. Astrophys. 639, A63 (2020).
- Gaulme, P., Jackiewicz, J., Appourchaux, T., Mosser, B., Astrophys. J. 785, 5 (2014).
Press Release and Media Appearances:
- Press Release article
- News Article in Göttinger Tageblatt (in German)
Butterfly diagrams of Kepler stars are unlike that of the Sun
At the start of a solar cycle sunspots appear on the Sun at latitudes of about ±35°, and as the cycle progresses they appear at lower latitudes. This time dependence of the latitudes of sunspot emergence translates into a time dependence of the rotation rates of sunspots because spots at different latitudes rotate at different rates (a consequence of solar differential rotation). The level of activity and rotation rates can be measured on a number of stars besides the Sun, and Nielsen et al. (2019) looked at the relationship between the phase of the activity cycle and the rotation rate of the magnetic features.
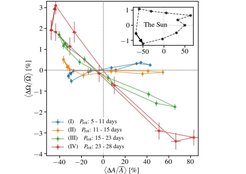
This work (Nielsen et al. 2019) used the sample of stars from Kepler that have known rotation rates and also have significant periodicity in their photometric variability (an indicator of magnetic activity) from Reinhold, Cameron & Gizon (2017). From this subset of 3203 stars, we removed the 110 stars that have periods very close to either 1 year or 185 days as these periodicities could be related to the instrument (e.g., associated with quarterly rolls of the telescope). For each of the remaining 3093 stars, we then measured the spot rotation rate of the star, Ω(t), and the activity level, A(t), as functions of time t with a cadence of 90 days. To be able to compare different stars, we considered the relative variations ΔΩ(t)/Ω and ΔA(t)/A̅, where temporal means are denoted with a bar. Since we know the mean period of rotation of the star, we phase-folded the inferred activity levels over the activity cycles. Up to this point, we analyzed each star separately. To improve the signal-tonoise ratio we then averaged over stars that have similar temperatures and rotation periods. Figure shows the average relationship between the relative variation of the rates at which the spots are rotating and the relative variation in the activity (where the average is over stars with similar periods). Systematic trends can be seen between stars with the different rotation rates. We also found that none of the groups of stars had a pattern similar to the Sun.
[Go back to Research Topic and Recent Result, or to the top of this page ]
References:
- Nielsen, M. B., Gizon, L., Cameron, R. H., Miesch, M., Astron. Astrophys. 622, A85 (2019).
- Reinhold, T., Cameron, R. H., Gizon, L., Astron. Astrophys. 603, A52 (2017).
Discovering small planets using a new transit detection algorithm
One of the main science objectives of the PLATO mission is the detection of Earth-sized planets in the habitable zones around Sun-like stars. After launch in 2026, PLATO will search for these planets by measuring the periodic small-amplitude brightness variations of their host stars that occur if a planet passes in front of its star, as seen from Earth. While a handful of Earth-sized planets have already been found in previous surveys using this so-called transit method, almost all of these planets were either in orbit around a small red dwarf star or they orbited a solar-type host star in a very close-in orbit with orbital periods of typically just a few days. The challenge in finding Earth analogs is in the scarcity of their transit events (once a year) and in the shallow signal. The transit depth in the stellar light curve is roughly given by the planet-to-star radius ratio squared. For an Earth-sized planet around a Sun-like star, the amplitude is only about 84 parts per million (ppm).
Detections of such small signals are only possible in the high-quality data from space missions such as the retired Kepler mission or the upcoming PLATO mission. Moreover, the odds of an Earth analog detection are much better for photometrically quiet stars compared to active stars. And finally, detection of a tiny signal can only be achieved using a sensitive search algorithm. Previous transit search algorithms were either extremely fast (in terms of computational speed) due to their simplified assumptions about the shape of the signal (e.g., assuming a box-like decrease of the stellar brightness in transit), or they were very accurate in terms of a modeling approach but their computational cost was so high that the algorithm was unsuitable for a large survey of transiting planets in thousands of light curves.
We have developed a new transit search algorithm that combines the good of both. Our Transit Least Squares (TLS) algorithm (Hippke & Heller 2019) looks for periodic variations in stellar photometry that can be described by a transit profile with realistic stellar limb darkening (Fig. Panel a). The resulting signal detection efficiency (SDE) is much higher than for the Box Least Squares (BLS) algorithm, which had served as a standard algorithm for transit searches for almost two decades (Kovács et al. 2002).
To test the benefits of TLS over previous transit search algorithms, we performed a transit survey in the archival data of the extended Kepler mission (K2). Instead of scanning the entirety of the hundreds of thousands of stellar light curves from K2, we focused on those systems that were known to have at least one transiting planet, 517 at that time. Our aim was to search for additional planets in these light curves that can be detected with TLS and that had been missed by previous searches.

In Heller et al. (2019a, 2019b) we reported the findings of a total of 18 new exoplanets in these light curves. Their transit signatures are typically 200 ppm deep (ranging from 100 ppm to 2000 ppm), and their orbital periods extend from about 0.7 d to 34 d with a median value of about 4 d. Fourteen of these 17 systems only had one known planet before, and so they joined the growing number of multi-planet systems. Most stars in our sample have subsolar masses and radii. In Fig. Panel b we show that our newly discovered planets are very small compared to most of the previously detected planets. These small planetary radii in our sample are a direct result of the higher signal detection efficiency of TLS compared to other search algorithms, proving TLS to be the state-of-the-art of transit detection algorithms. Thus, TLS marks a significant step in the development of transit search algorithms and towards finding Earth-like planets around Sun-like stars with PLATO.
[Go back to Research Topic and Recent Result, or to the top of this page ]
References:
- Heller, R., Rodenbeck, K., Hippke, M., Astron. Astrophys., 625, A31(2019a).
- Heller, R., Hippke, M., Rodenbeck, K., Astron. Astrophys. 627, A66 (2019b).
- Hippke, M., Heller, R., Astron. Astrophys. 623, A39 (2019).
- Kovács, G., Zucker, S., Mazeh, T., Astron. Astrophys. 391, pp. 369-377 (2002).
Distant star is roundest object ever observed in nature
Scientists measure the shape of a pulsating star with unprecedented precision.
November 16, 2016
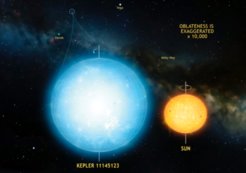
Stars are not perfect spheres, several mechanisms can change their shape. One mechanism is rotation: the more quickly a star rotates, the more flat it becomes due to the centrifugal force. Since distant stars appear as points in the sky, measuring their shape is a challenging task. A team of researchers led by Prof. Dr. Laurent Gizon from the Max Planck Institute for Solar System Research (MPS) and the University of Göttingen succeeded in measuring the oblateness of a slowly rotating star. In their study, which is published on 16 November, 2016 in the journal Science Advances, they determine for the first time stellar oblateness with unprecedented precision using asteroseismology – the study of the oscillations of stars. The technique is applied to a star 5000 light years (47,000,000 billion kilometers) away from Earth and reveals that the difference between the equatorial and polar radii of the star is only 3 kilometers – a number that is astonishing small compared to the star’s mean radius of 1.5 million kilometers.
References:
- Shape of a slowly rotating star measured by asteroseismology, Gizon, L. et al.: Science Advances, 2016 Nov; 2(11): e1601777.
Press Release:
Interior Rotation of a Distant Star Revealed (2013)
With the help of asteroseismic data obtained by the CoRoT space telescope, scientists were able to determine the interior rotation of a Sun-like star - and characterise an exoplanet.
2013
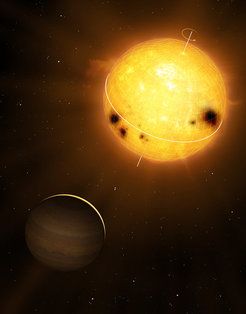
A team of scientists led by researchers from the Max Planck Institute for Solar System Research (Germany) and the University of Göttingen (Germany) have unambiguously measured the internal rotation of a Sun-like star and determined the inclination of its rotation axis for the first time. Their calculations show that the star rotates about 2.3 times faster than the Sun, with the axis of rotation inclined at 30 degrees to our line of sight. The star, HD52265, is located more than 90 light years away in the constellation of Monoceros. The results also prove that the body known to accompany the star is indeed an exoplanet and not - as previously argued - a small companion star called a brown dwarf. This is the first time that asteroseismology, the study of a star's internal oscillations, has been used to constrain the mass of an orbiting companion of a Sun-like star. The results were published in the journal "Proceedings of the National Academy of Sciences of the United States of America" (PNAS).
References
- Seismic constraints on rotation of Sun-like star and mass of exoplanet, Gizon et al., Proceedings of the National Academy of Sciences of the United States of America (PNAS), Early Edition, 29. Juli 2013