
Analyzing the Magnetic Field in the Solar Atmosphere
The magnetic field in the solar atmosphere exceeds the geomagnetic field strength by four orders of magnitude.
It greatly influences the processes of energy transport within the solar atmosphere, and dominates the morphology of the solar chromosphere and corona. Kinetic energy from convective motions in the Sun can be efficiently stored in magnetic fields and subsequently released - to heat the solar corona to several million degrees or to blast off coronal mass ejections.
The determination of the conditions in the lower layers of the solar atmosphere (photosphere - chromosphere), including the three-dimensional structure of the magnetic field therein, is the main task within this PhD project. Knowing these accurately is essential for many things, understanding turbulent magnetoconvection, finding out how energy is loaded onto the magnetic field lines that is then transported up to the corona and deposited there, uncovering the secrets of how heavy prominences can stay suspended in the thin corona, or gaining new insights into the solar cycle and solar irradiance variations.
MPS has access to world leading facilities to measure these conditions: space-based observatories like Solar Orbiter or Hinode, the largest available ground-based solar telescopes and the balloon-borne Sunrise observatory, deliver maps of the emitted solar radiation, displaying details down to scales of about 70 km. For the ground-based observatories SLAM group members have recently built or are building our own, often highly novel instruments, which provide us with outstanding and unique data.
Solar Orbiter: the Sun up close
The Solar Orbiter space mission, launched in February 2020, is the first mission ever to leave the ecliptic plane with optical instrumentation on board. Circling along its highly eccentric orbit around the Sun, Solar Orbiter provides both imaging and in-situ measurements of our star from viewpoints that are not Earth-based, allowing for novel science and novel techniques to be developed. Your task will be to contribute in making such potentials a successful reality.
The Polarimetric and Helioseismic Imager (SO/PHI) is an imaging spectro-polarimeter which provides both full-disk and high resolution vector-magetograms, Dopplergrams and continuum images of the Sun. The SO/PHI instrument has been built by an international consortium led by MPS.
Reaching distances from the Sun as small as 0.28AU, SO/PHI provides high-resolution vector magnetograms of exceptional quality. The near-synchronous rotation of Solar Orbiter with the Sun in some parts of its orbit will allow SO/PHI to track solar features for longer time than ever done so far from Earth-bound observatories. Last but not least, the stereoscopic possibilities offered by combining SO/PHI with other observatories open the door to new methodologies for studying the solar magnetic and velocity fields at all scales with a totally new perspective.
As an example of the novel methodologies exploiting the SO/PHI unique orbit, the combination of SO/PHI with Earth-bound observatories now allows for the full, solely observation-based determination of the photospheric vector magnetic field. Spectropolarimetric reconstructions of the photospheric vector magnetic field are intrinsically limited by the so-called 180-degree ambiguity, meaning that the field component perpendicular to the line of sight can be measured in intensity and direction, but its orientation remains undetermined. So far, the removal of such an ambiguity had required assumptions about the properties of the photospheric field. With SO/PHI is now possible to observe the same region on the sun from two different vantage points and to determine the true orientation of the transverse magnetic field component solely based on observations.
Depending on your interests, you could help on a wide range of projects, from developing data analysis tools for SO/PHI to the analysis and exploitation of the first science data of one of the major instruments on board Solar Orbiter.
As the leader team supporting the operation and science exploitation of PHI, we can offer you a research program that suits your interests, develops your skills and competences, gives you the possibility of wide and international collaborations, and boosts your chances of your future career in solar physics by having a head start in Solar Orbiter exciting science phase!
Unlocking the Sun's secrets with unique data from the balloon-borne observatory Sunrise
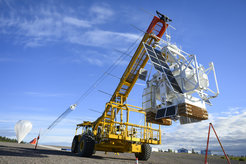
Sunrise is a balloon-borne solar observatory developed under the leadership of MPS. The 1-meter telescope is the largest solar telescope ever to leave the ground, allowing for highest spatial resolution measurements (~50 km on the Sun) in UV wavelength bands not accessible with ground-based observatories.
Two successful flights at an altitude of 37 km around the north pole have delivered a wealth of high-quality data sets, which have already resulted in immense new insights into the working of the solar photosphere (the layers of the solar atmosphere just at the solar surface), including a number of breakthroughs. These are documented in well over a hundred mostly high-impact scientific publications, making Sunrise by far the most successful balloon-borne mission to date. Currently, preparations are ongoing for the third flight of the Sunrise observatory, which will be even more powerful thanks to three new instruments that are being built for it. These include a unique and novel spectropolarimeter to make optimal use of the UV wavelength band. These new instruments will greatly expand the capabilities of Sunrise, enabling it to unlock not just hidden secrets of the photosphere, but also of the solar chromosphere, the most mysterious part of the solar atmosphere.
A third flight of the observatory unfortunately was not successful. A technical failure prevented us to obtain the anticipated high-quality data. We are currently preparing for a new launch attempt, ideally in June 2024.
The student will therefore enter the project in its most exciting phase, being involved in the preparation for the flight, the development of the scientific observing plan, laying out the theoretical basis for the successful data analysis after the flight. The PhD student will be directly involved in the first analysis of these data and will have a good chance of making/participating in the first discoveries from the Sunrise III data.
Also, a PhD with the correct skills and starting in 2023 has a chance of attending the launch from ESRANGE in north Sweden (2024) and participating in the operations of Sunrise during its flight in the stratosphere.
The Sun in 4D: "How to capture the properties of the solar atmosphere fast enough?"
![Example observation with a MiHI prototype at the Swedish 1-meter Solar Telescope [http://www.isf.astro.su.se/NatureNov2002/telescope_eng.html]. The red square on the left panel shows the spatial field of view of MiHI within a larger context scene of the solar surface. The small panels on the right show different cuts through the multi-dimensional data cube with spatial, spectral and polarization information recorded simultaneously with MiHI. Panels S1 to S4 show example spectra for the region around 6302 Å containing 2 spectral lines of neutral iron (denoted by ‘Fe I’ in panel S1). S1 shows an intensity (Stokes I) spectrum. Panels S2 to S4 show spectra of the degree of linear polarization (Stokes Q/I and U/I) and circular polarization (Stokes V/I). The horizontal axes represent the relative wavelength. Panels I1 to I4 show two-dimensional spatial images of the MiHI field of view in the 4 polarization states and spectrally averaged across a small region of the spectrum (denoted by the vertical yellow lines in panels S1 to S4). Panels C1 to C8 represent different spectral cuts through those images. The cuts have one spatial dimension and one spectral dimension. In cuts C1 to C4 the horizontal axis represents the spectral dimension. The vertical axis represents the spatial dimension cutting through the images along the blue lines. In cuts C5 to C8 the spectral dimension is vertical and the spatial dimension horizontal, cutting through the images along the red lines. The small structures seen in the images as well as in the Fe I lines in the spectral cuts are signatures of the Zeeman effect, which results from the interaction of the iron atoms with light in the presence of a magnetic field in the solar atmosphere.](/6268677/original-1567430564.jpg?t=eyJ3aWR0aCI6MjQ2LCJvYmpfaWQiOjYyNjg2Nzd9--747b78b4b4ac55e379eab261dc5159817cf89162)
Example observation with a MiHI prototype at the Swedish 1-meter Solar Telescope [http://www.isf.astro.su.se/NatureNov2002/telescope_eng.html]. The red square on the left panel shows the spatial field of view of MiHI within a larger context scene of the solar surface. The small panels on the right show different cuts through the multi-dimensional data cube with spatial, spectral and polarization information recorded simultaneously with MiHI. Panels S1 to S4 show example spectra for the region around 6302 Å containing 2 spectral lines of neutral iron (denoted by ‘Fe I’ in panel S1). S1 shows an intensity (Stokes I) spectrum. Panels S2 to S4 show spectra of the degree of linear polarization (Stokes Q/I and U/I) and circular polarization (Stokes V/I). The horizontal axes represent the relative wavelength. Panels I1 to I4 show two-dimensional spatial images of the MiHI field of view in the 4 polarization states and spectrally averaged across a small region of the spectrum (denoted by the vertical yellow lines in panels S1 to S4). Panels C1 to C8 represent different spectral cuts through those images. The cuts have one spatial dimension and one spectral dimension. In cuts C1 to C4 the horizontal axis represents the spectral dimension. The vertical axis represents the spatial dimension cutting through the images along the blue lines. In cuts C5 to C8 the spectral dimension is vertical and the spatial dimension horizontal, cutting through the images along the red lines. The small structures seen in the images as well as in the Fe I lines in the spectral cuts are signatures of the Zeeman effect, which results from the interaction of the iron atoms with light in the presence of a magnetic field in the solar atmosphere.
To study the dynamics of the continuously evolving solar atmosphere, it is necessary to obtain morphological information by imaging the solar surface on a time scale that is sufficiently small to effectively "freeze" the solar scene. With the European Solar Telescope - EST we expect to resolve solar structures of order 30 km which evolve at a time scale of seconds. While an image can be successfully recorded within this short time scale, only a small fraction of the information required for understanding the solar atmosphere is encoded in the image properties alone.
By analyzing in detail the strength, shape and the polarization state of the many spectral lines that are present in the solar spectrum, detailed information about the atmospheric stratification of temperature, vertical bulk motion, magnetic field strength and direction, encoded by atomic line transitions of the many elements that constitute the solar atmosphere, can be obtained. However, for a detailed analysis of the spectral properties of the Sun, a spectrograph with a high spectral resolution must be used.
There is currently no solar instrument in operation that can record spectral, polarization and spatial information within the limits of the short timescale of solar evolution that will be seen by the European Solar Telescope - EST, and with sufficient resolution and sensitivity. Present-day instruments are only able to capture all this information by scanning either spectrally or spatially which takes more time.
A new type of instrumentation is therefore needed for the EST. The Microlens-fed Hyperspectral Imager (MiHI) and the Helium Spectro-Polarimeter (HeSP), both under development at the Max Planck Institute for Solar System Research, are examples of such new instruments that overcome this problem by recording the spectral information for each image pixel simultaneously. In order to accomplish this, the pixel must first be reduced in size to create enough space on the two-dimensional image sensor for the spectral information to be dispersed without overlap with the neighboring pixels. The microlens spectrograph uses an assembly of microlens arrays to reduce the pixel size and create space for the spectral information, while minimizing the loss of light. Then the light is dispersed in a spectrograph, forming an array of spectra on the detector.
A fair amount of data from MiHI is ready to be analyzed. A unique opportunity to work on unique data in an environment where both, instrumental and scientific expertise, meet on a daily basis.